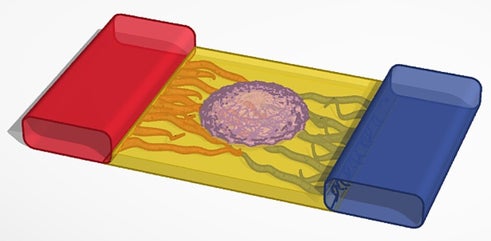
Shear Stress in Organ-on-a-Chip Systems
Shear stress is a force exerted by fluid moving over a surface. Let’s have a look at the shear stress inside a microfluidic channel.
Liquid in straight microfluidic channels flows in layers that rub against each other tangentially. Viscosity (internal friction) is one of the phenomena of fluid to resist the movement of one of their parts relative to another in the flow. The outermost layer of liquid rubs tangentially against the solid walls of the microfluidic channel on which cells can grow. Thus, the shear stress is the force exerted by fluid moving over the surface of the microfluidic channel.
The shear stress is the force per unit area exerted on walls of a microfluidic channel by fluid flowing next to these walls. In organ-on-a-chip devices, this stress is essential because it mimics the mechanical stimuli experienced by cells in the body, particularly in blood vessels, lungs, and other tissues exposed to dynamic fluid environments. Proper control over shear stress can lead to more accurate cellular behavior, simulating real-world physiological conditions, which is critical for disease modeling, drug testing, and tissue engineering.
Length of a channel did not affect velocity and shear stress within microchannels. However, it has been shown that channels with sharp turns have regions of near-zero velocity at the turns, leading to the accumulation and adhesion of large numbers of cells. This non-uniform adhesion, especially in the turn regions compared to other parts of the channel, combined with high variability in cell adhesion, indicates that channels with sharp turns are not suitable for applications that require predictable results. In contrast, channels with curved turns offer more consistent and predictable cell adhesion. The level of cell adhesion in these curved channels is similar to that seen in straight channels without turns. However, straight channels have rectangular corners where walls are meeting creating a defined and abrupt physical transition.
Different cross-sectional geometries can lead to different shear stress profiles. In microfluidic channels with straight corners shear stress acts differently compared to more rounded geometries like circular channels. In a rectangular channel, the distribution of shear stress is not uniform. Instead, it exhibits a gradient that can affect how cells respond within the channel. Understanding and controlling this gradient is crucial for applications like cell culture and drug testing in organ-on-a-chip models. Let’s explore shear stress distribution inside a rectangular microfluidic channel.
Rectangular channels are widely used in organ-on-a-chip systems, particularly in simulating organs like the BBB, lung, liver, and gut, where shear stress impacts cells differentiation. Rectangular channels, due to their straightforward geometry, offer a clear view of how shear stress varies from the channel straight corners to walls center parts.
The formula for shear stress 𝜏 𝑤 in a rectangular channel is:
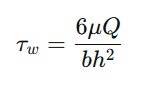
Where:
- 𝜇 is the dynamic viscosity of the fluid,
- 𝑄 is the volumetric flow rate,
- 𝑏 is the channel width,
- ℎ is the channel height.
These channels typically have a small height-to-width ratio. This means that the height of the channel is lower than its width. That is very useful for chip demolding during manufacturing and makes them ideal for cell monolayers, as cells can be exposed to physiologically relevant shear stress levels, simulating blood or air flow.
Balanced and more uniform shear stress across the entire channel is required in some organ-on-a-chip system, making them suitable for cells that grow well under smooth mechanical stimuli. However, corners of channels tend to create not uniform shear stress across walls making it difficult for ensuring accurate modeling of in vivo like physiological conditions in organ-on-a-chip systems. The paper "Organ-on-a-Chip: Design and Simulation of Various Microfluidic Channel Geometries for the Influence of Fluid Dynamic Parameters" explores the effect of shear stress gradients inside microfluidic channels. It simulates different geometries and analyzes how parameters like channel width, height, and flow rate influence velocity and shear stress distributions COMSOL Multiphysics (COMSOL Inc., version 5.5, 2020, Stockholm, Sweden).
In this study, a longitudinal rectangular microchannel (Figure 2a) is designed to investigate shear stress and velocity profile distribution under three different flow rates, along with the channel width (Figure 6a-i). Simulated shear stress and velocity are evaluated using three different flow rates: 2, 6 and 10 µL/m at the bottom of the microchannel (h = 0 µm- solid lines) and at the middle of the microchannel (h = 100 µm- dotted line).
The simulation results demonstrate:
- the fluid moves parallel to the walls, with the highest velocity at the center parts of the walls and a minimum within the corners;
- a uniform distribution of the shear stress within the microchannel;
- velocity profile has a parabolic shape with near-zero velocity within the corners;
- the highest shear stress occurs near the central parts of the walls with near-zero level within the corners, creating a gradient that cells will experience differently depending on their location.
The findings from the study highlight how small adjustments in channel geometry and flow parameters can significantly influence the shear stress landscape inside the device affecting cell behavior and function. This is particularly valuable for designing devices tailored to specific organs or physiological systems.
Strategies to manage shear stress in rectangular microfluidic channel
Rectangular channels are best for simulating tissue layers or organs like the BBB, gut, liver, and lungs, where shear stress needs to be applied over a flat surface. When designing such organ-on-a-chip systems, choosing the right channel geometry is essential to replicating the physiological shear stress conditions necessary for accurate tissue and organ function. Here are some tips for optimizing the design of rectangular microfluidic channels:
- Corner rounding
In organ-on-a-chip microphysiological systems, near-zero shear stress in the corners may cause abnormal behavior which could influence cells alignment, function, and gene expression. Chamfered or rounded corners eliminate near-zero areas of shear stress allowing fluid to flow more smoothly along the channel walls. This helps in preventing shear stress peaks and improves fluid flow consistency.
- Aspect ratio optimization
By adjusting the aspect ratio of the channel (the ratio between width and height), shear stress distribution can be optimized to minimize extreme shear stress values at the walls and corners.
- Flow rate control
Reducing the flow rate decreases the overall shear stress in the channel. This can be critical in systems designed for sensitive biological applications like organ-on-a-chip.
Understanding and mitigating shear stress in cornered channels is essential for ensuring accurate modeling of physiological conditions in organ-on-a-chip devices.
Conclusion
Shear stress plays a fundamental role in the design and function of organ-on-a-chip microphysiological systems. The cross-sectional geometry of microfluidic channels directly impacts how shear stress is distributed, influencing everything from cell behavior and nutrient transport to drug testing outcomes. By carefully selecting the appropriate channel geometry, researchers can optimize organ-on-a-chip systems to more accurately replicate human physiology, making these devices indispensable for drug discovery, disease modeling, and personalized medicine.
If you are working with organ-on-a-chip systems or interested in microfluidic devices with rectangular channels, feel free to explore our PimCell ® microfluidic chips and accessories. All devices can be customized for your particular research needs.
Visit our websites www.pimcell.com and www.pimbio.com for more information.